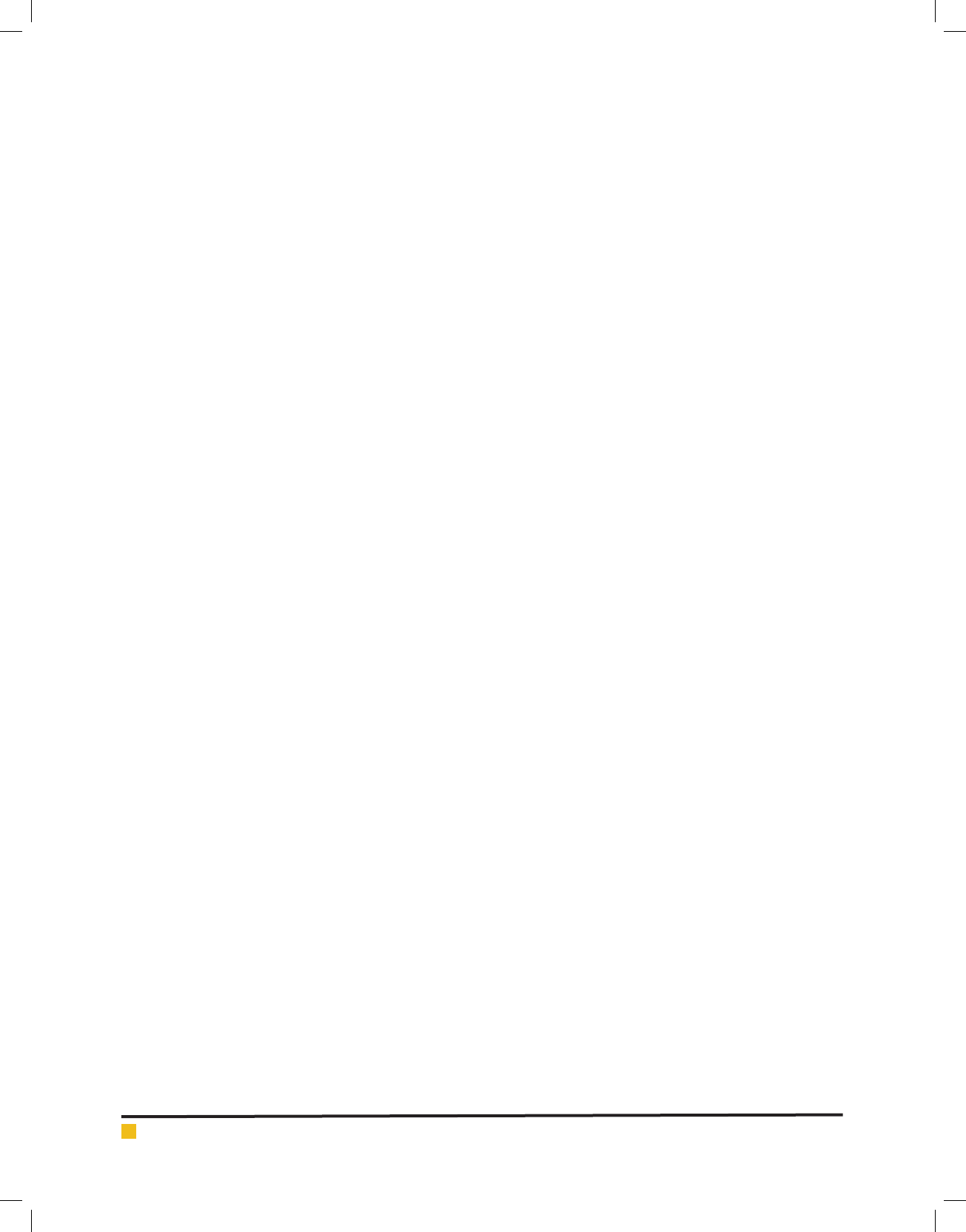
642 MANIPULATING DISEASE AND PEST RESISTANCE PATHWAYS IN PLANTS BIOSCIENCE BIOTECHNOLOGY RESEARCH COMMUNICATIONS
Paramita Ghosh et al.
Jirschitzka J, Mattern DJ, Gershenzon J, D’Auria JC. (2013)
Learning from nature: new approaches to the metabolic engi-
neering of plant defense pathways. Current Opinion in Bio-
technology 24, 320-328.
Jongedijk E, Tigelaar H, Vanroekel JSC, Bresvloemans SA, Dek-
ker I, Vandenelzen PJM, Cornelissen BJC, Melchers LS. (1995)
Synergistic activity of chitinases and beta-1,3-Glucanases
enhances fungal resistance in transgenic tomato plants.
Euphytica 85:173-80.
Jordá L, Conejero V, Vera P (2000) Characterization of P69E
and P69F , two differentially regulated genes encoding new
members of the subtilisin-like proteinasi family from tomato
plants. Plant Physiology 122:67–74.
Kazuko Yamaguchi-Shinozaki and Kazuo Shinozaki (2005)
Organization of cis-acting regulatory elemens in osmotic and
cold -stress-responsive promoters. Trends in plant science. Vol.
10 No.2.
Keller H, Pamboukdjian N, Ponchet M, Poupet A, Delon R, Ver-
rier JL, et al. (1999) Pathogen-induced elicitin production in
transgenic tobacco generates a hypersensitive response and
nonspeci c disease resistance. Plant Cell 11:223-35.
King SRF, McLellan H, Boevink BC, Armstrong MR, Bukharova
T, Sukarta O, Win J, Kamoun S, Birch PRJ, Ban eld MJ. (2014)
Phytophtora infestans RXLR effector PexRD2 interacts with
host MAPKKKe to suppress plant immune signaling. Plant
Cell.113.120055.
Kostov K, Christova P, Slavov S, Batchvarova R. (2009) Consti-
tutive expression of a radish defensin gene Rs-Afp2 in tomato
increases the resisstance to fungal pathogens. Biotechnology &
Biotechnological Equipment 23:1121-5.
Kumar V, Parkhi V, Kenerley CM, Rathore KS. (2009) Defence-
related gene expression and enzyme activities in transgenic
cotton plants expressing an endochitinase gene from Tricho-
derma virens in response to interaction with Rhizoctonia
solani. Planta 230:277-91.
Kunkel BN, Brooks DM. (2002) Cross talk between signaling
pathways in pathogen defence. Current Opinion in Plant Biol-
ogy 5:325-331.
Lee SK, song MY, Seo YS, Kim HK, Ko S, et al.(2009) Rice Pi5-
mediated resistance to Magnaporthe oryzae requires the pres-
ence of two CC-NBLRR genes. Genetics 181:1627-1638.
Li HP, Zhang JB, Shi RP, Huang T, Fischer R, Liao YC. (2008)
Engineering fusarium head blight resistance in wheat by
expression of a fusion protein containing a fusarium-speci c
antibody and an antifungal peptide. Molecular Plant-Microbe
Interactions 2008; 21:1242-8.
Li J, Brader G, Palva ET. (2004) The WRKY70 transcription fac-
tor: A node of convergence for jasmonate-mediated and salic-
ylate-mediated signals in plant defence. Plant Cell 16:319-31.
Li J, Brader G, Kariola T, Palva ET (2006) WRKY70 modulates
the selection of signaling pathways in plant defence. The Plant
Journal 46:477-491.
Lin WC, Lu CF, Wu JW, Cheng ML, Lin YM, Yang NS,
Black L,
Green SK, Wang JF, Cheng CP.
(2004) Transgenic tomato plants
expressing the Arabidopsis NPR1 gene display enhanced resist-
ance to a spectrum of fungal and bacterial diseases. Transgenic
Research 13:567-81.
Liu T, Liu Z, Song C, Hu Y, Han Z, She J, Fan F, Wang J, Jin C,
Chang J, Zhou JM, Chai J. (2012) Chitin-induced dimerization
activates a plant immune receptor. Science 336:1160-1164.
Loutre C, Wicker T, Travella S, Galli P, Sco eld S, et al. (2009)
Two different CC-NBS-LRR genes are required for Lr10 –medi-
ated leaf rust resistance in tetraploid and hexaploid wheat.
Plant Journal 60: 1043-1054.
Magdalena Rossi,Fiona L. Goggin,Stephen B. Milligan,Isgouhi
Kaloshian,Diane E. Ullman, and Valerie M. Williamson (1998)
The nematode resistance gene Mi of tomato confers resistance
against the potato aphid. Proceedings of the National Academy
of Sciences USA 95:9750–9754.
Makandar R, Essig JS, Schapaugh MA, Trick HN, Shah J. (2006)
Genetically engineered resistance to Fusarium head blight in
wheat by expression of Arabidopsis NPR1. Molecular Plant-
Microbe Interactions 19:123-9.
Maldonado AM, Doerner P, Dixon RA, Lamb CJ, Cameron RK.
(2002) A putative lipid transfer protein involved in systemic
resistance signaling in Arabidopsis. Nature 419:399-403.
Mari Narusaka, Kubo Y, Hatakeyama K, Imamura J, Ezura H,
Nanasato Y, Tabei Y, Takano Y, Shirasu K, Narusaka Y.(2013)
Interfamily Transfer of Dual NB-LRR Genes Confers Resistance
to multiple pathogens. . Public Library of Science (PLOS) one
8:e55954.
Meur G, Budatha M, Srinivasan T, Kumar KRR, Gupta AD,
Kirti PB. (2008) Constitutive expression of Arabidopsis NPR1
confers enhanced resistance to the early instars of Spodoptera
litura in transgenic tobacco. Physiologia Plantarum 133:765-
75.
Michelmore, R.W. (2003) The impact zone: genomics and
breeding for durable disease resistance. Current Opinion in
Plant Biology 6:397–404.
Molla KA,Karmakar S,Chanda PK,Sarkar SN,Datta SK,Datta
K (2016). Tissue-speci c expression of Arabidopsis NPR1 gene
in rice for sheath blight resistance without compromising phe-
notypic cost. Plant Sci 250:105-114.
Mur LA, Kenton P, Atzorn R, Miersch O and Wasternack C.
(2006) The outcomes of concentration include synergy, antag-
onism, and oxidative stress leading to cell death. Plant Physi-
ology 140:249-262.
Ndamukong I, Al Abdallat A, Thurow C, Fode B, Zander M,
Weigel R, Gatz C. (2007) SA-inducible Arabidopsis glutare-
doxin interacts with TGA factors and suppresses JA-responsive
PDF1.2 transcription. The Plant Journal 50:128-39.
Nelson R, Wiesner-HanksT, Wisser R and Balint-Kurti P (2017)
Navigating complexity to breed disease-resistant crops. Nature
Reviews Genetics (Advanced publication)
Nombela, G., Williamson, V. M., & Muñiz, M. (2003). The root-
knot nematode resistance gene Mi-1.2 of tomato is responsible
for resistance against the white y Bemisia tabaci. Molecular
Plant-Microbe Interactions 16(7):645-649.