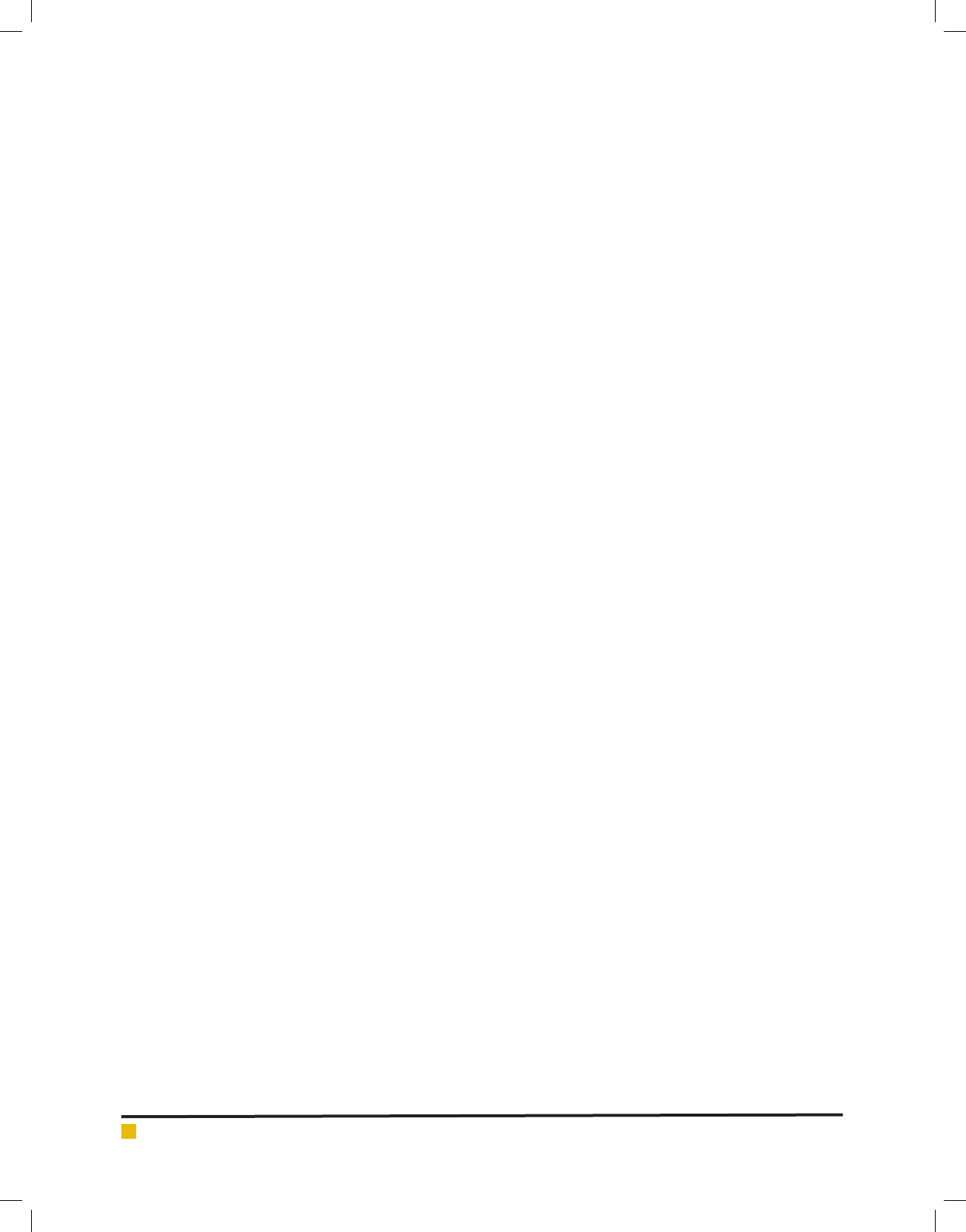
Madhurjya Gogoi et al.
206
IN-SILICO
IDENTIFICATION OF PHYTOHORMONE PATHWAY GENES BIOSCIENCE BIOTECHNOLOGY RESEARCH COMMUNICATIONS
Gaille, C., Reimmann, C. and Haas, D. (2003) Isochorismate
Synthase (PchA), the First and Rate-limiting Enzyme in Salicy-
late Biosynthesis of Pseudomonas aeruginosa. J. Biol. Chem.,
278(19), 16893-16898. doi: 10.1074/jbc.m212324200.
Gamalero, E. and Glick, B. R. (2012) Ethylene and abiotic
stress tolerance in plants. In: P. Ahmad and M. N. V. Prasad
(eds) Environmental Adaptations and Stress Tolerance of
Plants in the Era of Climate Change. New York: Springer, pp.
395-412.
doi: 10.1007/978-1-4614-0815-4_18
Gao, Q.-M., Zhu, S., Kachroo, P. and Kachroo, A. (2015) Signal
regulators of systemic acquired resistance. Front. Plant Sci.,
06. doi: 10.3389/fpls.2015.00228.
Hammond-Kosack, K. E. and Jones, J. D. G. (2000) Response to
plant pathogens. In: B., Gruissem, W. Buchannan, and R. Jones
(eds)Biochemistry and molecular biology of plants. Rockville,
MD: American Society of Plant Physiologists, Wiley, pp. 1102-
1157.
Harms, K., Atzorn, R., Brash, A., Kuhn, H., Wasternack, C.,
Willmitzer, L. and Pena-Cortes, H. (1995) Expression of a Flax
Allene Oxide Synthase cDNA Leads to Increased Endogenous
Jasmonic Acid (JA) Levels in Transgenic Potato Plants but Not
to a Corresponding Activation of JA-Responding Genes. The
Plant Cell, 7(10), p. 1645. doi: 10.2307/3870026.
Horváth, E., Szalai, G. and Janda, T. (2007) Induction of Abiotic
Stress Tolerance by Salicylic Acid Signaling. J Plant Growth
Regul, 26(3), 290-300. doi: 10.1007/s00344-007-9017-4.
Huang, D., Wu, W., Abrams, S. R. and Cutler, A. J. (2008) The
relationship of drought-related gene expression in Arabidop-
sis thaliana to hormonal and environmental factors. Journal
of Experimental Botany, 59(11), 2991-3007. doi: 10.1093/jxb/
ern155.
Khan, M. I. R., Fatma, M., Per, T. S., Anjum, N. A. and Khan,
N. A. (2015) Salicylic acid-induced abiotic stress tolerance and
underlying mechanisms in plants. Front. Plant Sci., 6. doi:
10.3389/fpls.2015.00462.
Koornneef, A. and Pieterse, C. M. (2008) Cross Talk in
Defense Signaling. Plant Physiology, 146(3), 839-844. doi:
10.1104/107.112029.
Koornneef, A., Leon-Reyes, A., Ritsema, T., Verhage, A., Den
Otter, F. C., Van Loon, L. and Pieterse, C. M. (2008) Kinetics
of Salicylate-Mediated Suppression of Jasmonate Signaling
Reveal a Role for Redox Modulation. Plant Physiology, 147(3),
1358-1368. doi: 10.1104/108.121392.
Lu, S., Su, W., Li, H. and Guo, Z. (2009) Abscisic acid improves
drought tolerance of triploid Bermuda grass and involves
H2O2- and NO-induced antioxidant enzyme activities. Plant
Physiology and Biochemistry, 47(2), 132-138. doi: 10.1016/j.
plaphy.2008.10.006.
Melotto, M., Underwood, W., Koczan, J., Nomura, K. and He, S.
Y. (2006) Plant Stomata Function in Innate Immunity against
Bacterial Invasion. Cell, 126(5), 969-980. doi: 10.1016/j.
cell.2006.06.054.
Mittler, R. and Blumwald, E. (2010) Genetic Engineering for
Modern Agriculture: Challenges and Perspectives. Annu. Rev.
Plant Biol., 61(1), 443-462. doi: 10.1146/annurev-arplant-
042809-112116.
Mittler, R. and Blumwald, E. (2015) The Roles of ROS and ABA
in Systemic Acquired Acclimation. Plant Cell, 27(1), 64-70.
doi: 10.1105/tpc.114.133090.
Miura, K. and Tada, Y. (2014) Regulation of water, salinity, and
cold stress responses by salicylic acid. Front. Plant Sci., 5. doi:
10.3389/fpls.2014.00004.
Niki, T., Mitsuhara, I., Seo, S., Ohtsubo, N. and Ohashi, Y.
(1998) Antagonistic Effect of Salicylic Acid and Jasmonic Acid
on the Expression of Pathogenesis-Related (PR) Protein Genes
in Wounded Mature Tobacco Leaves. Plant and Cell Physiol-
ogy, 39(5), 500-507. doi: 10.1093/oxfordjournals.pcp.a029397.
O’Donnell, P. J., Calvert, C., Atzorn, R., Wasternack, C., Leyser,
H. M. O. and Bowles, D. J. (1996) Ethylene as a Signal Mediat-
ing the Wound Response of Tomato Plants. Science, 274(5294),
1914-1917. doi: 10.1126/science.274.5294.1914.
Osakabe, Y., Yamaguchi-Shinozaki, K., Shinozaki, K. and Tran,
L.-S. P. (2013) ABA control of plant macro element membrane
transport systems in response to water de cit and high salin-
ity,New Phytol., 202(1), 35-49. doi: 10.1111/nph.12613.
Pal, M., Szalai, G., Kovacs, V., Gondor, O. K. and Janda, T.
(2013) Salicylic acid-mediated abiotic stress tolerance. In: S.
Hayat, A. Ahmed, and M. N. Alyemeni (eds) Salicylic Acid
Plant Growth and Development. Rotterdam, Netherlands:
Springer, pp. 183–247. doi: 10.1007/978-94-007-6428-6_10
Preston, C. A., Lewandowski, C., Enyedi, A. J. and Bald-
win, I. T. (1999) Tobacco mosaic virus inoculation inhibits
wound-induced jasmonic acid-mediated responses within
but not between plants. Planta, 209(1), 87-95. doi: 10.1007/
s004250050609.
Qin, X. and Zeevaart, J. A. D. (1999) The 9-cis-epoxycarot-
enoid cleavage reaction is the key regulatory step of absci-
sic acid biosynthesis in water-stressed bean. Proceedings of
the National Academy of Sciences, 96(26), 15354-15361. doi:
10.1073/pnas.96.26.15354.
Raghavendra, A. S., Gonugunta, V. K., Christmann, A. and
Grill, E. (2010) ABA perception and signaling. Trends in Plant
Science, 15(7), 395-401. doi: 10.1016/j.tplants.2010.04.006.
Rejeb, I., Pastor, V. and Mauch-Mani, B. (2014) Plant Responses
to Simultaneous Biotic and Abiotic Stress: Molecular Mecha-
nisms. Plants, 3(4), 458-475. doi: 10.3390/plants3040458.
Riemann, M., Dhakarey, R., Hazman, M., Miro, B., Kohli, A. and
Nick, P. (2015) Exploring Jasmonates in the Hormonal Network
of Drought and Salinity Responses. Front. Plant Sci., 6. doi:
10.3389/fpls.2015.01077.
Rivas-San Vicente, M. and Plasencia, J. (2011) Salicylic acid
beyond defence: its role in plant growth and development.
Journal of Experimental Botany, 62(10), 3321-3338. doi:
10.1093/jxb/err031.
Rouhier, N. and Jacquot, J.-P. (2008) Getting sick may help
plants overcome abiotic stress. New Phytol, 180(4), 738-741.
doi: 10.1111/j.1469-8137.2008.02673.x.