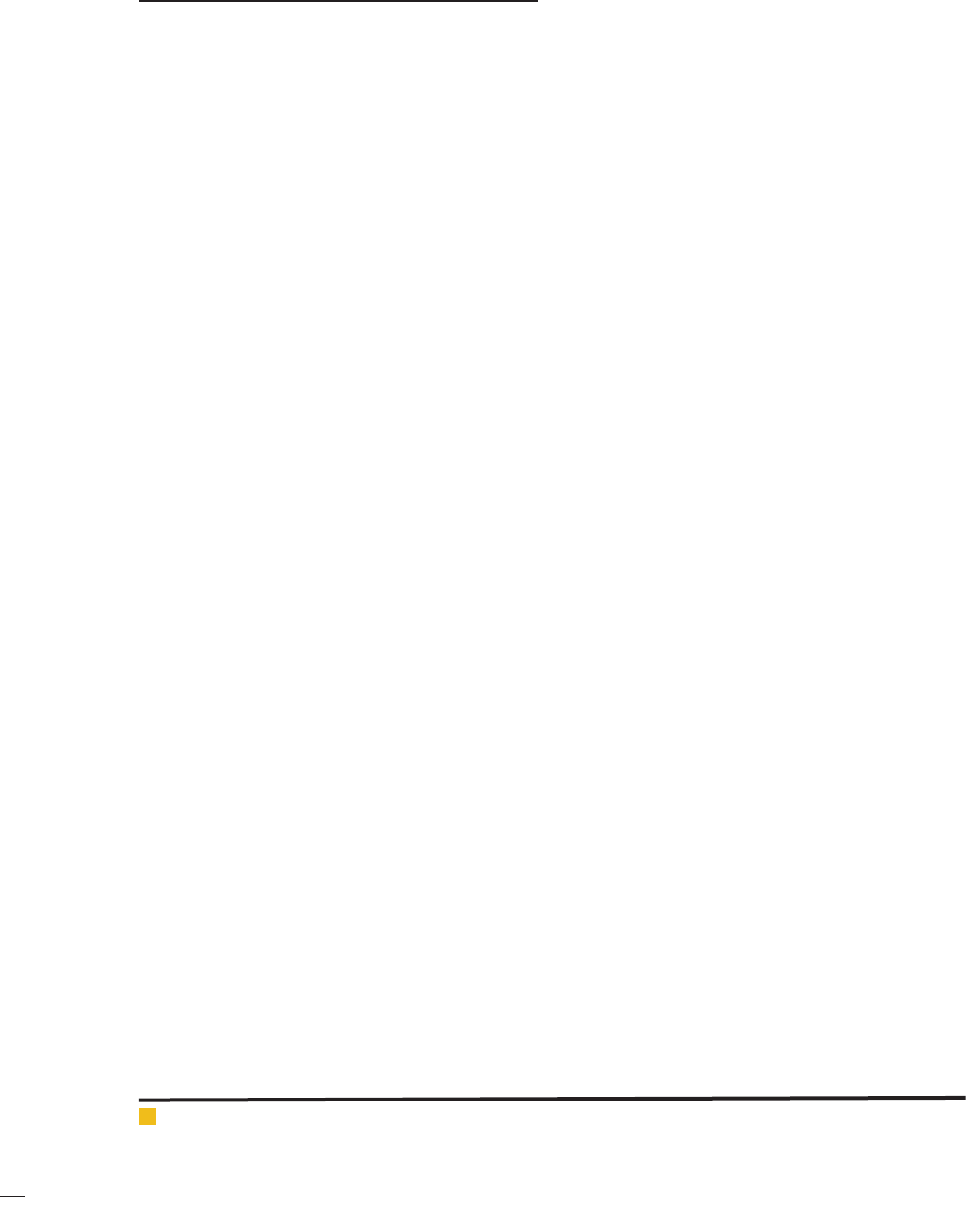
Majid Valizadeh et al.
826 EFFECTS OF MELATONIN ON REPAIR OF DNA DOUBLE STRAND BREAKS BIOSCIENCE BIOTECHNOLOGY RESEARCH COMMUNICATIONS
ACKNOWLEDGEMENT
This research was supported by Tehran University of
Medical Sciences in Iran with health services grant
No.28688.
REFERENCES
Ahnesorg P., Smith P., Jackson S.P. (2006). XLF interacts with
the XRCC4-DNA ligase IV complex to promote DNA nonho-
mologous end-joining. Cell, 124(2):301-13.
Azzam E., Jay-Gerin J.P., Pain, D. (2012). Ionizing radiation-
induced metabolic oxidative stress and prolonged cell injury.
Cancer Letters, 327(0): 48–60.
Bassing C.H., Chua K.F., Sekiguchi J. (2002). Increased ionizing
radiation sensitivity and genomic instability in the absence of
histone H2AX. Proceedings of the National Academy of Sci-
ences of the United States of America, 99(12):8173-8.
Brandsma I., Gent D.C. (2012). Pathway choice in DNA double
strand break repair: observations of a balancing act. Genome
Integrity, 3(1):9.
Cassatt D.R., Fazenbaker C.A., Bachy C.M., Hanson M.S. (2002).
Preclinical modeling of improved amifostine (Ethyol) use in
radiation therapy. Seminars in Radiation Oncology, 12(1 Suppl
1):97-102.
Ciccia A., Elledge S.J. (2010). The DNA damage response:
making it safe to play with knives. Molecular Cell, 40(2):179-
204
Chapman J.R., Taylor M.R., Boulton S.J. (2012). Playing the
end game: DNA double-strand break repair pathway choice.
Molecular Cell, 47(4):497-510.
Guirouilh-Barbat J., Huck S., Bertrand P. (2004). Impact of the
KU80 pathway on NHEJ-induced genome rearrangements in
mammalian cells. Molecular Cell, 14 (5): 611–23.
Hall E.J., Giaccia A.J. (2006). Radiobiology for the Radiologist.
6th. Lippincott Williams & Wilkins, Philadelphia, PA.
Hussein M.R. , Abu-Dief E.E. , Abd El-Reheem M.H. , Abd-
Elrahman, A. (2005). Ultrastructural evaluation of the radio-
protective effects of melatonin against X-ray-induced skin
damage in Albino rats. International Journal of Experimental
Pathology, 86(1):45–55.
Jobert A., Vogin G., Devic C., Granzotto A., Viau M., Maalouf
M. (2011). Radiation biology: major advances and perspectives
for radiotherapy]. Cancer radiotherapie: Journal De La Societe
Francaise De Radiotherapie Oncologique, 15(5):348-54 . (Arti-
cle in French)
Koc M., Taysi S., Buyukokuroglu M.E., Bakan N. (2003). Mela-
tonin protects rat liver against irradiation-induced oxidative
injury. Journal Of Radiation Research, 44(3):211-5.
Lieber M.R. (2010). The mechanism of double-strand DNA
break repair by the nonhomologous DNA end joining pathway.
Annual Review Of Biochemistry, 79:181-211
Lieber M.R. (2008). The mechanism of human nonhomologous
DNA end joining. Journal of Biological Chemistry, 283:1–5.
Lomax M.E., Folkes L.K., O’Neill P. (2013). Biological Conse-
quences of Radiation-induced DNA Damage: Relevance to
Radiotherapy. Clinical Oncology,25(10):578-85.
Mao Z., Bozzella M., Seluanov A., Gorbunova V. (2008). Com-
parison of nonhomologous end joining and homologous recom-
bination in human cells. DNA Repair (Amst), 7(10):1765–1771.
Mohseni M., Mihandoost E., Shirazi A., Sepehrizadeh Z., Baz-
zaz J.T., Ghazi-khansari M. (2012). Melatonin may play a role
in modulation of bax and bcl-2 expression levels to protect rat
peripheral blood lymphocytes from gamma irradiation-induced
apoptosis. Mutation Research/Fundamental and Molecular
Mechanisms of Mutagenesis, 738(2):19-27.
Parihar V.K., Dhawan J., Kumar S., Manjula S., Subramanian
G., Unnikrishnan M. et al. (2007). Free radical scavenging and
radioprotective activity of dehydrozingerone against whole
body gamma irradiation in Swiss albino mice. Chemico-bio-
logicalinteractions, 170(1):49-58.
Prasad KN. (1995). Handbook of radiobiology (2nd Ed.), CRC
Press, Boca Raton, FL.
Reiter R.J. (1991). Pineal melatonin: cell biology of its synthe-
sis and of its physiological interactions. Endocrine Reviews,
12(2):151-80.
Rezaeejam H., Shirazi A., Valizadeh M., Izadi A. (2015). Candi-
date gene biodosimeters of mice and human exposure to ioniz-
ing radiation by quantitative reverse transcription polymerase
chain reaction. Journal of Cancer Research and Therapeutics,
11(3): 549-557.
Rodriguez C., Mayo J.C., Sainz R.M., Antolin I., Herrera F.,
Martín V. (2004). Regulation of antioxidant enzymes: a sig-
ni cant role for melatonin. Journal Of Pineal Research, 36(1):
1-9.
San Filippo J., Sung P., Klein H. (2008). Mechanism of eukary-
otic homologous recombination. Annual Review Of Biochem-
istry,77:229-57.
Schulte-Uentrop L., El-Awady R., Schliecker L., Willers H.,
Dahm-Daphi J. (2008). Distinct roles of XRCC4 and Ku80 in
non-homologous end-joining of endonuclease-and ionizing
radiation-induced DNA double-strand breaks. Nucleic Acids
Research, 36(8):2561-9.
Shirazi A., Mihandoost E., Mohseni M., Ghazi-Khansari M.,
Rabie Mahdavi S. (2011). Radio-protective effects of melatonin
against irradiation-induced oxidative damage in rat peripheral
blood. Physica Medica, 29(1):65-74.
Shirazi A. (2011). Radioprotective effect of melatonin in reduc-
ing oxidative stress in rat lenses. Cell Journal, 13(2):79.
Shirazi A., Haddadi G., Minaee B., Sepehrizadeh Z., Mahdavi
S., Jaberi E. (2010). Evaluation of melatonin for modulation
of apoptosis-related genes in irradiated cervical spinal cord.
International Journal of Low Radiation,7(6):436-45.
Shrivastav M., De Haro L.P., Nickoloff J.A. (2008). Regula-
tion of DNA double-strand break repair pathway choice. Cell
Research, 18(1):134-47.
Thompson L.H., Schild D. (2001). Homologous recombinational
repair of DNA ensures mammalian chromosome stability.